Explore this page
Program Staff
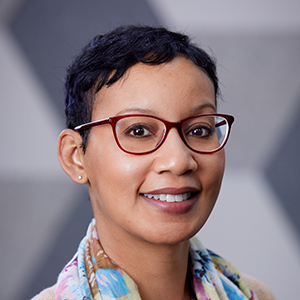
Stephanie A. Morris, Ph.D.
- Program Lead, CEGS Program
- Division of Genome Sciences
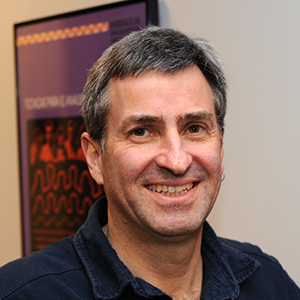
Mike Pazin, Ph.D.
- Program Director
- Division of Genome Sciences
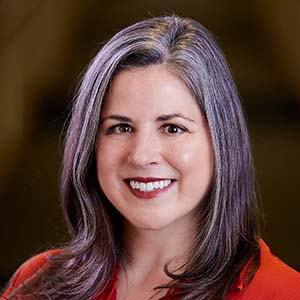
Lisa H. Chadwick, Ph.D.
- Deputy Director
- Division of Genome Sciences
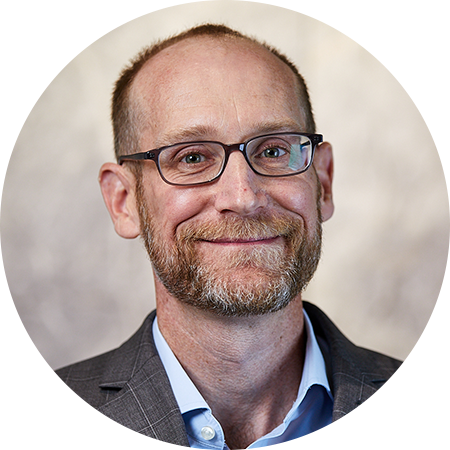
Daniel A. Gilchrist, Ph.D.
- Program Director
- Division of Genome Sciences
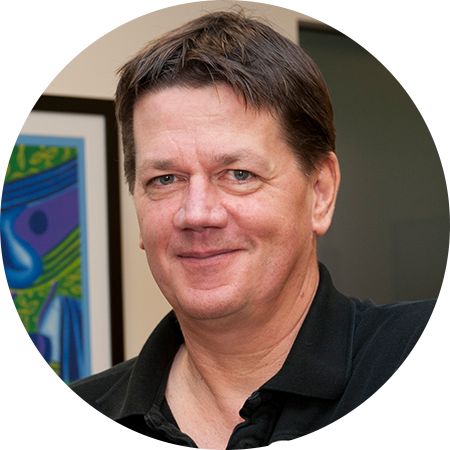
Colin Fletcher, Ph.D.
- Program Director, The Knockout Mouse Project (KOMP)
- Division of Genome Sciences
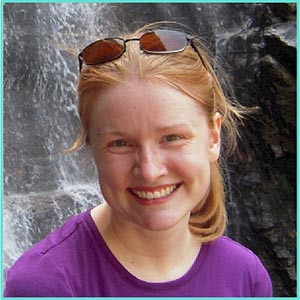
Mollie Minear, Ph.D.
- Program Director
- Division of Genomic Medicine
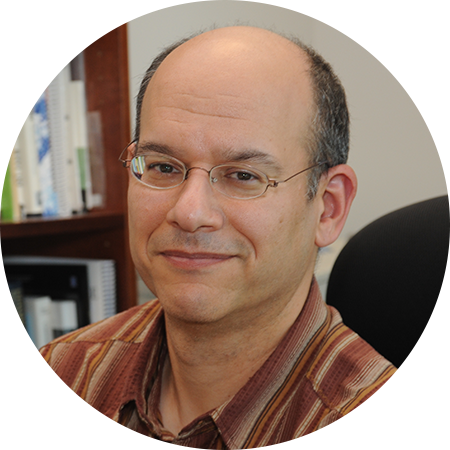
Adam Felsenfeld, Ph.D.
- Program Director
- Extramural Programs Branch
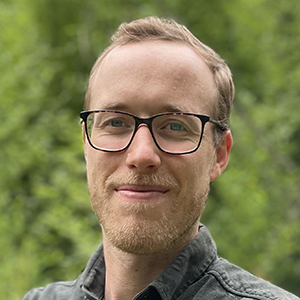
Ian C. Nova, Ph.D.
- Program Director
- Division of Genome Sciences
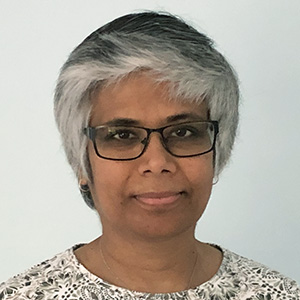
Sandhya Xirasagar, Ph.D.
- Program Director
- Office of Genomic Data Science
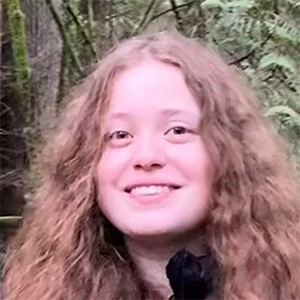
Alessandra L. Serrano Marroquin, B.A.
- Scientific Program Analyst
- Division of Genome Sciences
Last updated: February 27, 2025